Quantum Entropy: Exploring the Dynamics of Thermal Speed
Written on
Chapter 1: The Realm of Quantum Phase Transitions
Imagine a universe where the conventional rules of physics do not apply. Here, quantum fluctuations take center stage, leading to sudden and dramatic shifts in the state of matter. This is the domain of quantum phase transitions, where the very essence of reality can transform in an instant. At the core of this phenomenon lies the concept of dynamical quantum phase transitions (DQPTs). Unlike classical transitions that occur in a state of equilibrium, DQPTs transpire out of equilibrium, presenting a captivating puzzle for researchers. Investigating these transitions paves the way for a deeper understanding of the universe's intricate systems and their evolution over time.
The Geometry of Quantum Entropy
One of the most intriguing aspects of DQPTs is the geometric framework used to analyze entropy production. Consider quantum states as points within an expansive, multidimensional space. The distance between these points can be measured through a concept known as the Bures angle. During a DQPT, the Bures angle between a system's initial and evolved states increases rapidly, signaling a surge in entropy production. This geometric viewpoint offers a fresh way to visualize and measure the transformations occurring during these transitions. By charting these geometric changes, scientists can anticipate the behavior of quantum systems under varying conditions.
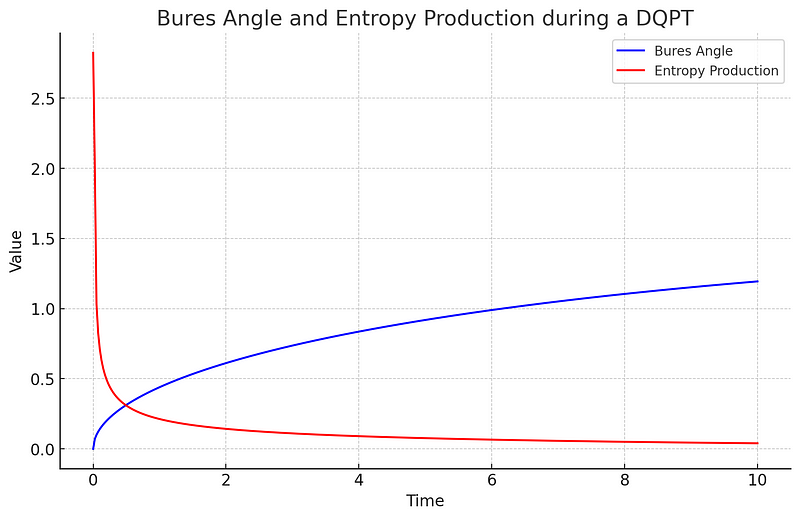
The graph above illustrates the relationship between the Bures angle and entropy production during a dynamical quantum phase transition. The Bures angle is represented in blue, while entropy production is shown in red, demonstrating how both evolve over time.
Implications for Future Technologies
The exploration of DQPTs extends beyond theoretical inquiry; it holds tangible implications for technology. For example, comprehending how entropy is generated and controlled in quantum systems could lead to breakthroughs in quantum computing. These advanced machines utilize quantum mechanics principles to process information at speeds far beyond classical computers. By leveraging the rapid entropy production linked to DQPTs, we could devise more efficient algorithms and error-correction techniques. Furthermore, insights from DQPT research could guide the creation of innovative materials with unique properties, paving the way for advancements in energy storage, telecommunications, and beyond.
Entropy and Irreversibility
Entropy serves as a measure of disorder and irreversibility within a system. When a quantum system undergoes a DQPT, the acceleration of entropy production underscores the irreversible nature of these transitions. This swift increase in disorder is a defining characteristic that sets DQPTs apart from classical phase transitions.
Bures Angle and Quantum States
The Bures angle quantifies the distance between quantum states geometrically. During a DQPT, this angle experiences significant growth, indicating a substantial transformation in the system's state. This geometric approach aids in visualizing and quantifying the changes in entropy production.
Quantum Fluctuations Drive Change
In contrast to classical phase transitions driven by temperature variations, quantum phase transitions are propelled by quantum fluctuations. These fluctuations prompt sudden and dramatic shifts in the state of matter, resulting in the intricate dynamics observed in DQPTs.
Link to the Second Law of Thermodynamics
The relationship between DQPTs and the second law of thermodynamics is groundbreaking. It affirms that even in the quantum landscape, the principle remains valid: entropy, or disorder, consistently increases in an isolated system. This insight bridges classical and quantum thermodynamics.
Applications in Quantum Computing
The principles underlying DQPTs may be applied to quantum computing, potentially leading to more effective algorithms and error-correction methods. Understanding how entropy is generated and managed in quantum systems is vital for progressing this cutting-edge technology.
The Dawn of a Quantum Future
As we delve deeper into the world of dynamical quantum phase transitions, we unveil a universe brimming with potential and complexity. The rapid acceleration of entropy production in these systems not only challenges our grasp of thermodynamics but also unlocks opportunities for revolutionary technologies. Envision a future where quantum computers tackle problems in seconds that would take classical computers millennia, where new materials with unprecedented properties reshape industries, and where our understanding of the universe's fundamental processes expands beyond imagination. The study of DQPTs illuminates a path of hope and inspiration, heralding a future where the impossible becomes achievable.
Chapter 2: Quantum Fluctuations and Energy
In this chapter, we will explore the impact of quantum fluctuations on energy production and efficiency, offering insights into the potential for unlimited energy sources.
The first video delves into the concept of quantum tunneling and its potential to unlock unlimited energy, providing a fascinating glimpse into the future of energy generation.
The second video examines how quantum entanglement influences entropy, shedding light on the intricate relationships within quantum systems.