# Understanding the Nature of Electron Beams in Quantum Physics
Written on
Chapter 1: The Enigma of Wave-Particle Duality
In the realm of quantum mechanics, one of the most perplexing ideas is the concept of wave-particle duality. Standard physics texts often assert that light exhibits a dual nature: behaving as both a particle and a wave, depending on the situation. This notion extends to electrons, which are also considered to be both particles and waves simultaneously. However, we contend that this interpretation may be misleading and stems from outdated ways of conceptualizing physical entities.
Our aim in this article is to address the question: what constitutes an electron beam?
Most educational materials describe electrons as minuscule particles that orbit around a positively charged nucleus, creating an electric current when they move through conductors. While this explanation is straightforward and easy to grasp, it raises an important question: how do we know this to be true? Have scientists actually observed tiny spheres moving within conductors when voltage is applied? The answer is “NO.” This portrayal arises from interpretations of experiments where no one has witnessed a point-like electron, largely because, if electrons were indeed spherical, their size would be too small to observe using light.
To grasp the nature of an electron, consider this simple experiment: direct a laser pointer towards a wall and activate it. You will see merely a spot of light on the wall. To visualize the light beam itself, you need to introduce smoke into its path. This allows you to observe a straight luminous beam emanating from the laser pointer and striking the wall.
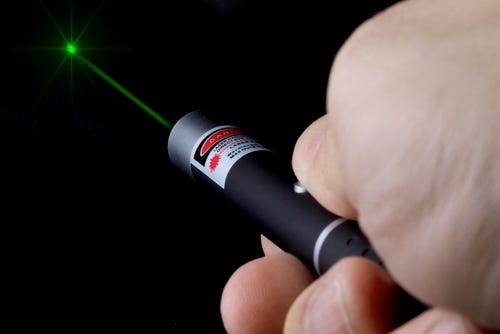
When you shine a laser beam on a wall, the luminous spot is all you notice; the beam itself remains invisible (the beam in this image has been digitally enhanced). The only way to observe the light beam is by introducing smoke, which scatters the light particles, making them visible to your eyes. The light perceived is not directly from the laser beam heading towards the wall; rather, it is the light that has been deflected towards your eyes by interacting with the smoke particles.
In a similar vein, when observing students in a classroom, you don’t see them directly; you see light reflected off their surfaces. If you doubt this, turn off the lights and close the blinds. Can you still see anyone? Likely not. However, would you conclude that the students have vanished simply because they are no longer visible?
Lesson 1: Our perception is effectively a scattering experiment where light acts as a projectile. The light that reaches our eyes is deflected by objects, and our brains construct an image based on the distribution of light.
Repeating the experiment with a diffraction grating placed in the light beam reveals its wave characteristics, resulting in an interference pattern, famously demonstrated in Young’s double-slit experiment.
Now, let’s replicate this experiment using a beam of electrons instead of light. An electron beam can be generated by heating a wire within a high-voltage capacitor. The elevated voltage accelerates these charged particles, similar to the mechanics of cathode-ray tubes, as illustrated below.
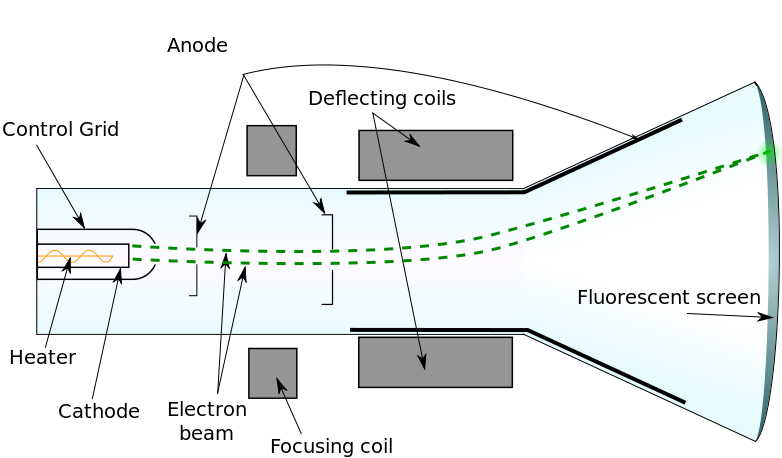
Inside the cathode-ray tube, nothing is visible, akin to the case of the laser beam directed at the wall. However, when the beam strikes a fluorescent screen, it creates a visible green spot. Are we witnessing electrons? Indeed! If we see students illuminated by the light they emit, we are observing electrons emitting light from their interactions. How can we ascertain that these emissions originate from electrons rather than just light? The answer is straightforward: the beam can be deflected by a magnetic field. By adjusting the intensity and direction of the magnetic field, the luminous spot on the fluorescent screen shifts accordingly due to the Lorentz force. This indicates that the beam is electrically charged, but does it confirm that the beam consists of particles? Not necessarily.
Lesson 2: An electron beam behaves similarly to a light beam, with the primary distinction being that the electron beam is electrically charged, as evidenced by its deflection in a magnetic field.
We can conduct a similar experiment with electrons by filling the tube with gas. This allows us to visualize the beam, as what we actually observe is the light scattered by gas atoms that the electron beam strikes. Below is a depiction of a cathode-ray tube filled with nitrogen, placed within a uniform magnetic field.
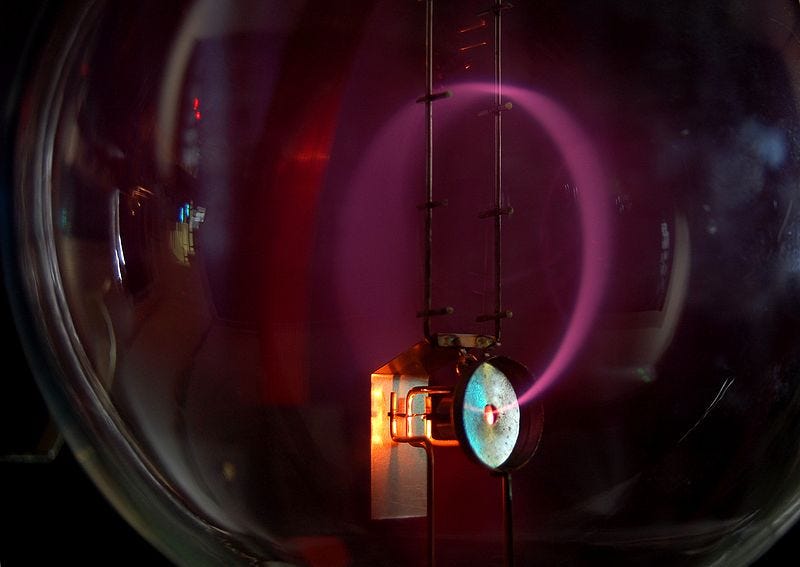
Finally, we can place a diffraction grating along the path of the electron beam. In this scenario, the grating must have a very low slit spacing, achievable, for instance, with a graphite crystal. The result is a diffraction pattern displayed on the fluorescent screen:
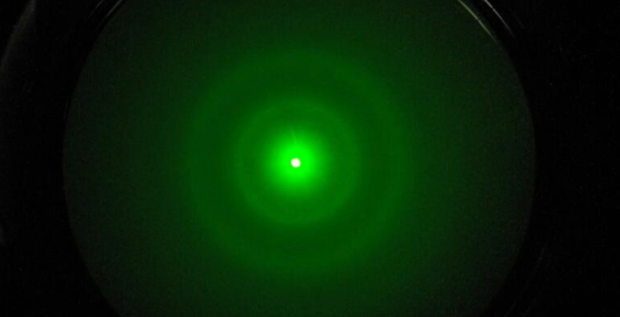
The distance between the interference fringes varies with the energy of the beam. Therefore, we can conclude that an electron beam comprises waves emitted from the cathode, traveling through the tube. These waves carry an electric charge, meaning they do not have to propagate in straight lines and can interact with materials to produce light.
Lesson 3: An electron beam propagates as a wave, resulting in diffraction phenomena.
Moreover, electron microscopes utilize electron beams in a manner analogous to how optical microscopes use light, allowing for the focusing of electron beams with Einzel lenses, just as light can be focused with convergent lenses.
Electromagnetism teaches us that light consists of electromagnetic fields propagating as waves. Hence, the most intuitive conclusion from our experiments is that an electron beam is essentially a charged field propagating in wave form.
The objective of this discussion was to encourage a perspective that considers electrons primarily as waves. We do not need to delve into quantum mechanics for this understanding. Future discussions will explore the concept of a charged field and delve into the particle aspects of such fields.
To further enhance your understanding of quantum mechanics, check out the following video:
In this video, Chad Orzel aims to demystify quantum mechanics, providing insights that complement our discussion.
Additionally, another informative video is available:
In this presentation by Flavio Del Santo, the focus is on developing a measurement theory within quantum field theory, adding depth to our exploration of quantum phenomena.